- 1Laboratory in Microbiology and Marine Biotechnology, Department of Biotechnology, School of Biological Sciences, Alagappa University, Karaikudi, India
- 2Parasitology and Entomology Unit, Department of Microbiology and Infectious Diseases, French Armed Forces Biomedical Research Institute, Marseille, France
- 3IRD, SSA, AP-HM, VITROME, Aix-Marseille University, Marseille, France
- 4IHU Méditerranée Infection, Marseille, France
- 5National Reference Center for Malaria, Marseille, France
- 6Structural Biology and Bio-Computing Laboratory, Department of Bioinformatics, Alagappa University, Karaikudi, India
Since the rapid spread of coronavirus disease (COVID-19) became a global pandemic, healthcare ministries around the world have recommended specific control methods such as quarantining infected peoples, identifying infections, wearing mask, and practicing hand hygiene. Since no effective treatment for COVID-19 has yet been discovered, a variety of drugs approved by Food and Drug Administration (FDA) have been suggested for repurposing strategy. In the current study, we predicted that doxycycline could interact with the nucleotide triphosphate (NTP) entry channel, and is therefore expected to hinder the viral replication of SARS-CoV-2 RNA-dependent RNA-polymerase (RdRp) through docking analysis. Further, the molecular dynamics results revealed that the RdRp-Doxycycline complex was structurally relatively stable during the dynamic period (100 ns), and its complex maintained close contact with their active catalytic domains of SARS-CoV-2 RdRp. The molecular mechanics Poisson–Boltzmann surface area (MM-PBSA) calculation of binding free energy also showed that the doxycycline has worthy affinities with SARS-CoV-2 RdRp. As expected, doxycycline effectively inhibited the viral replication of IHU strains of SARS-CoV-2 (IHUMI-3 and IHUMI-6), identified from the hospitalized patients in IHU Méditerranée Infection (IHUMI), Marseille, France. Moreover, doxycycline inhibited the viral load in vitro at both on-entry and after viral entry of IHU variants of SARS-CoV-2. The results suggest that doxycycline exhibits strains-dependant antiviral activity against COVID-19. As a result, the current study concludes that doxycycline may be more effective in combination with other drugs for better COVID-19 treatment efficacy.
Introduction
Coronavirus disease 2019 (COVID-19) is a respiratory illness associated with signs such as a dry cough, fever, shortness of breath, muscle aches, fatigue, and dyspnea, leading to pneumonia (Wu et al., 2020). Acute respiratory distress syndromes (ARDS) such as hypercytokinemia, severe acute respiratory syndrome, lymphopenia, disseminated intravascular coagulation, renal failure, and mortality can occur in severe condition (Gostic et al., 2020). In severe situations, lung inflammation becomes so severe that it produces septic shock, which results in mortality owing to a decrease in blood pressure and oxygen deprivation. The pathognomonic symptoms as well as severity of COVID-19 vary from patient to patient (Menni et al., 2020; Shah et al., 2020). The current outbreak of COVID-19 was declared a global emergency of international concern by the WHO due to its rapid spread, with a measured basic reproductive number (Ro) of 2.2 (Rafiq et al., 2020). As of 10 August 2021, the WHO reports that 203,295,170 cases have been definitively diagnosed around the world, including 4,303,515 deaths.
COVID-18 has no specific treatment available at this time. Because COVID-19 has the potential to be a devastating disease for the entire civilization, healthcare authorities around the world have recommended a number of preventative measures, such as quarantining infected patients, wearing proper masks, regular hand sanitization on a regular basis, and rapid testing and diagnosis of those suspected of being infected (Li et al., 2020). Simultaneously, scientists are examining COVID-19 vaccines and therapeutic treatments (Pawar, 2020). However, novel medicines on the other hands require months, if not years, to reach the commercial market (Abd El-Aziz and Stockand, 2020). As a result, researchers are now developing a repurposing strategy to identify appropriate therapeutics among currently available drugs, to be used against COVID-19 (Phadke and Saunik, 2020).
Severe acute respiratory syndrome coronavirus 2 (SARS-CoV-2) is a positive-sense enveloped and single-strand RNA virus, which belongs to the Coronaviridae family and Betacoronavirus genus (Andreani et al., 2020). The novel SARS-CoV-2 is highly similar to SARS-CoV and MERS, and the genome size is approximately 30 kb (Zhang and Holmes, 2020; Zou et al., 2020). Among the SARS-CoV-2 viral proteins, the 3-chymotrypsin like protease (3CLpro) is an imperative enzyme that plays a fundamental role in the dispensation of viral polyproteins, which are necessary for viral development and their pathogenesis. Impeding 3CLpro activity could hinder the viral replication of SARS-CoV-2 and has been seen as a promising strategy for inventing suitable therapeutic agents against COVID-19 (Rathnayake et al., 2020). As a result, Owen et al. (2021) have recently reported that PF-07321332, an orally accessible SARS-CoV-2 main protease inhibitor, showed excellent antiviral activity with in vivo safety outline against COVID-19. Similarly, RNA-dependent RNA-polymerase (RdRp), a decisive polymerase enzyme that is crucial in the replication process, has been seen as a promising drug target for discovering potent antiviral agents against COVID-19 (Gao et al., 2020).
Due to the lack of a COVID-19 therapy, a variety of existing drugs are being used to treat the symptoms of patients with COVID-19 in the form of a drug-repurposing approach. Many more drugs, like antimicrobial, are now undergoing human trials to determine their efficacy against COVID-19 (Cimolai, 2020). Andreani et al. (2020) have reported that the antibacterial agent azithromycin showed synergistic anti-SARS-CoV-2 activity combined with hydroxychloroquine. Furthermore, Gautret et al. (2020) also demonstrated that the results of the combination of hydroxychloroquine and azithromycin in human trials were positive; all patients taking the combination were virologically restored to health within 6 days of treatment. In this study, we identified doxycycline as a potent antiviral compounds for SARS-CoV-2 through in silico and in vitro approach (Figure 1).
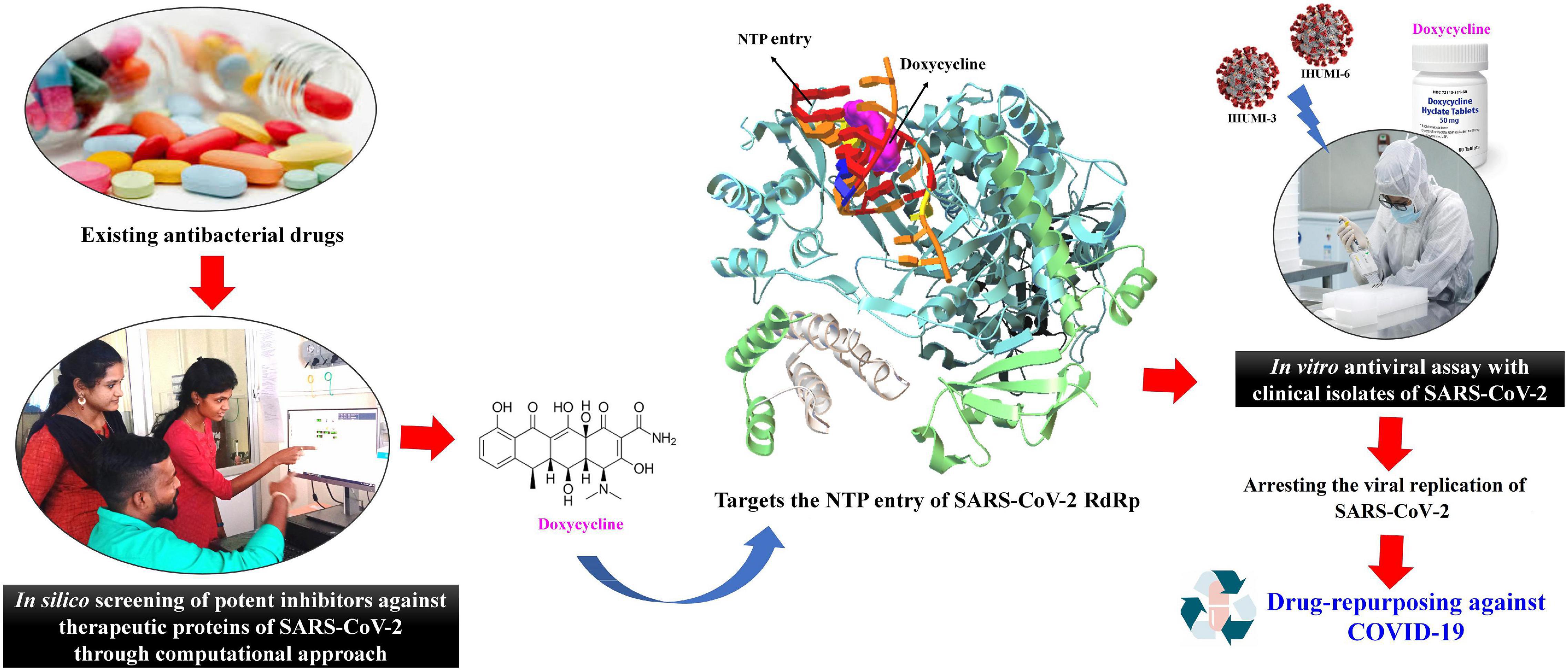
Figure 1. Graphical representation of the present study for the drug-repurposing of doxycycline against COVID-19.
Materials and Methods
Ligand Preparation
Drug profile of the existing antibacterial drugs (321 drugs) was obtained from the DrugBank server1. The canonical SMILES of the antibacterial drugs were obtained from the PubChem server. Then, the PDB format three-dimensional (3D) structure of the antibacterial drugs was generated using the Online SMILES Translator server (Alexpandi et al., 2019).
Protein Preparation
The 3D structure of SARS-CoV-2 RdRp-Nsp7-Nsp8 complex (PDB ID: 7AAP) was downloaded from the RCSB PDB database. The homology-modeled protein of SARS-CoV-2 RdRp was obtained from Prof. Hua Li, HUST, China (Wu et al., 2020). Energy minimization was performed using YASARA software and protein preparation was performed using the AutoDock Tools Version 1.5.6 (Balasubramaniam et al., 2019). The natural ligands present in the protein structures were isolated by deleting atomic orchestrates of the PDB file. During protein preparation, all the water molecules were deleted, and hydrogen polarities and Kollman charges were allocated, using AutoDock Tools Version 1.5.6 platform. Protein structures were then translated from PDB file to PDBQT file format to perform the docking analysis with AutoDock vina.
Molecular Docking
First, the top binding compounds with targeted proteins were scrutinized by virtual screening using the iGEMDOCK tool. After the finding of best compounds, the binding mode of top-scoring compounds with the active site of SARS-CoV-2 RdRp protein was studied using AutoDock Vina (Alexpandi et al., 2020). Ligands were independently docked with the therapeutic proteins adopting a flexible mode under rigid conditions. The grid size was set at 126 × 126 × 126 (x, y, and z) points with a grid spacing 1 Å, and the grid center was set based on the proteins. The docking was performed with AutoDock vina and prepared file was saved in PDBQT format. The binding scores of ligands were obtained in the form of Kcal/mol calculated using the Autodock vina scoring algorithm. The output of the docking files was then saved in PDB format for post-docking analysis. Post-docking analysis, including monitoring interacted amino acid residues, was performed using Maestro (Schrödinger) software. The predicted drugs in the present study were then compared with reported anti-COVID-19 agents such as lopinavir and remdesivir triphosphate (Cheng et al., 2020).
Molecular Dynamic Simulation Studies
To confirm the stability of the ligand-bound complexes of therapeutic targets of SARS-CoV-2, molecular dynamic simulation studies were performed for 100 ns using Gromacs software. The ligand topologies were obtained from the PRODRUG server. All ligand-bound structures were solvated with SPC216 water molecules and periodic boundary conditions were applied to all directions of cubic boxes with 1.0 and 1.2 nm dimensions. To obtain the precise electrostatic values during the dynamics, all of the systems (net charge) were neutralized with appropriate Na+ ions. Steepest Descent Algorithm (SDA) and Conjugate Gradient Algorithm (CGA) were used to perform energy minimization (50,000 steps) with a tolerance of 1,000 kJ mol–1 nm–1, followed by a two-step equilibrium phase (NPT-particles, volume and temperature; NVT-particles, pressure and temperature combinations). Bond constraints of the molecular simulations were maintained by the LINear Constraint Solver (LINCS) algorithm. The Particle Mesh Eshwald (PME) method was used to compute the long-range Coulomb interactions with the default cut-off parameter of 0.12 nm. Temperature (310 K) was regulated using the V-rescale weak coupling method and the Parrinello–Rahman (PR) method was used to equilibrate [pressure (1 atm), temperature, density, and total energy] the systems. All the pre-equilibrated systems were subjected to molecular dynamic simulation for 100 ns and the coordinates were saved every 2 ps (Choubey et al., 2020).
MM-PBSA Calculation for Protein-Ligand Complexes
In order to calculate the binding-free energies (affinities) of the complexes during the dynamics, MM-PBSA calculation was performed. Here, the trajectories obtained from last 10 ns were considered to calculate Gibbs free energy using g_mmpbsa tool (combined in the GROMACS software) and the following equation (Mariadasse et al., 2020).
Where, ΔGcomplex, is defined as total energy, ΔGprotein and ΔGligand are defined to reach the total binding-free energy of the protein and ligand.
In vitro Confirmation of Anti-SARS-CoV-2 Activity
Drugs, the Virus and Cells
Stock solutions of doxycycline hyclate (Sigma Aldrich, St Quentin Fallavier, France) were prepared in methanol and diluted in Minimum Essential Media (MEM, Gibco, ThermoFischer, Waltham, MA, United States), resulting in seven final concentrations ranging from 0.1 to 100 μM. Chloroquine diphosphate (Sigma Aldrich) and remdesivir (Apollo Scientific, Manchester, United Kingdom) were used as positive anti-SARS-CoV-2 compounds. Stock solutions of chloroquine were prepared in water and remdesivir in DMSO/water 10%. In total two clinically-isolated SARS-CoV-2 strains (IHUMI-3 and IHUMI-6), collected in hospitalized patients during the first COVID-19 outbreak in March 2020 in IHU Méditerranée Infection (IHUMI), Marseille, France, were maintained in production in Vero E6 cells (American type culture collection ATCC CRL-1586) in MEM with 4% fetal bovine serum and 1% glutamine (complete medium) (Gautret et al., 2020). All the experiments with SARS-CoV-2 were performed in the Biosafety Level (BSL)-3 Laboratory.
In vitro Antiviral Assay
Briefly, 96-well plates were prepared with 104 cells/mL of Vero E6 (200 μL per well), as previously described (Andreani et al., 2020). Doxycycline concentrations were added 4 h before infection. Vero E6 cells were infected with IHUMI-3 or IHUMI-6 strains at an MOI (multiplicity of infection) of 0.8. Reverse transcription polymerase chain reaction (RT-PCR) was used 48 h post-infection to estimate the replication using the Superscript III platinum one step with the Rox kit (Invitrogene) after extraction with the BIoExtract SuperBall kit (Biosellal, Dardilly, France). In the RT-PCR system for SARS-CoV-2 detection, the envelope protein (E)-encoding gene was used. The following primers and probe were used: E_forward (ACAGGTACGTTAATAGTTAATAGCGT), E_probe (FAM-ACACTAGCCATCCTTACTGCGCTTCG-TAMRA), E_reverse (ATATTGCAGCAGTACGCACACA) (Corman et al., 2020). The percentage of inhibition of SARS-CoV-2 replication was estimated for each drug concentration as follows:
(mean CTdrug concentration − mean CTcontrol 0%)/(mean CTcontrol 100% − mean CTcontrol 0%) × 100.
EC50 (median effective concentration) and EC90 (90% effective concentration) were calculated with the inhibitory sigmoid Emax model, which estimated the EC50 and EC90 through non-linear regression by using a standard function of the R software (ICEstimator version 1.2). The EC50 and EC90 values resulted in a mean of 7–10 independent experiments.
Determination of the Inhibition Stage
Furthermore, to identify the inhibition stage of doxycycline on the entry and post-entry of SARS-CoV-2 was evaluated with IHUMI-3 strain at a concentration of 10 μM. For the “full-time treatment,” Vero E6 cells were infected with the IHUMI-3 strain for 48 h after pre-incubation of the cells with the tested drugs for 4 h. For the “entry” treatment, the cells were infected for 2 h after pre-incubation for 4 h and then the virus-drug mixture was replaced with fresh medium maintained for 46 h. For the “post-entry” treatment, the cells were infected for 2 h and then incubated with drug for 46 h. The percentage of inhibition of SARS-CoV-2 replication by 10 μM of drug was estimated for each drug concentration as following:
(mean CTdrug concentration − mean CTcontrol 0%)/(mean CTcontrol 100% − mean CTcontrol 0%) × 100.
Results
Screening of Potent Inhibitors for SARS-CoV-2 RdRp
In the finding of SARS-CoV-2 RdRp-inhibitors, the existing antibacterial drugs such as amphomycin, vancomycin, malacidin A, doxycycline, natamycin, enviomycin, tyrothricin, colistin, tylosin, and lorvotuzumab mertansine were identified as the top binding drugs for RdRp (Figure 2 and Supplementary Table 1). Among the top-10 compounds, only the antibacterial drug doxycycline (−7.3 Kcal/mol) could inhibit the activity of SARS-CoV-2 RdRp by interacting with the NTP entry channel (Figure 3), as like remdesivir triphosphate (−7.8 Kcal/mol) (Supplementary Figure 1). The NTP entry channel is composed of hydrophilic amino acids, including Lys545, Arg553, and Arg555 (Figure 3). The results reveal that doxycycline has a similar binding site at SARS-CoV-2, similar to parental nucleotides with almost similar binding energy (Figure 3).
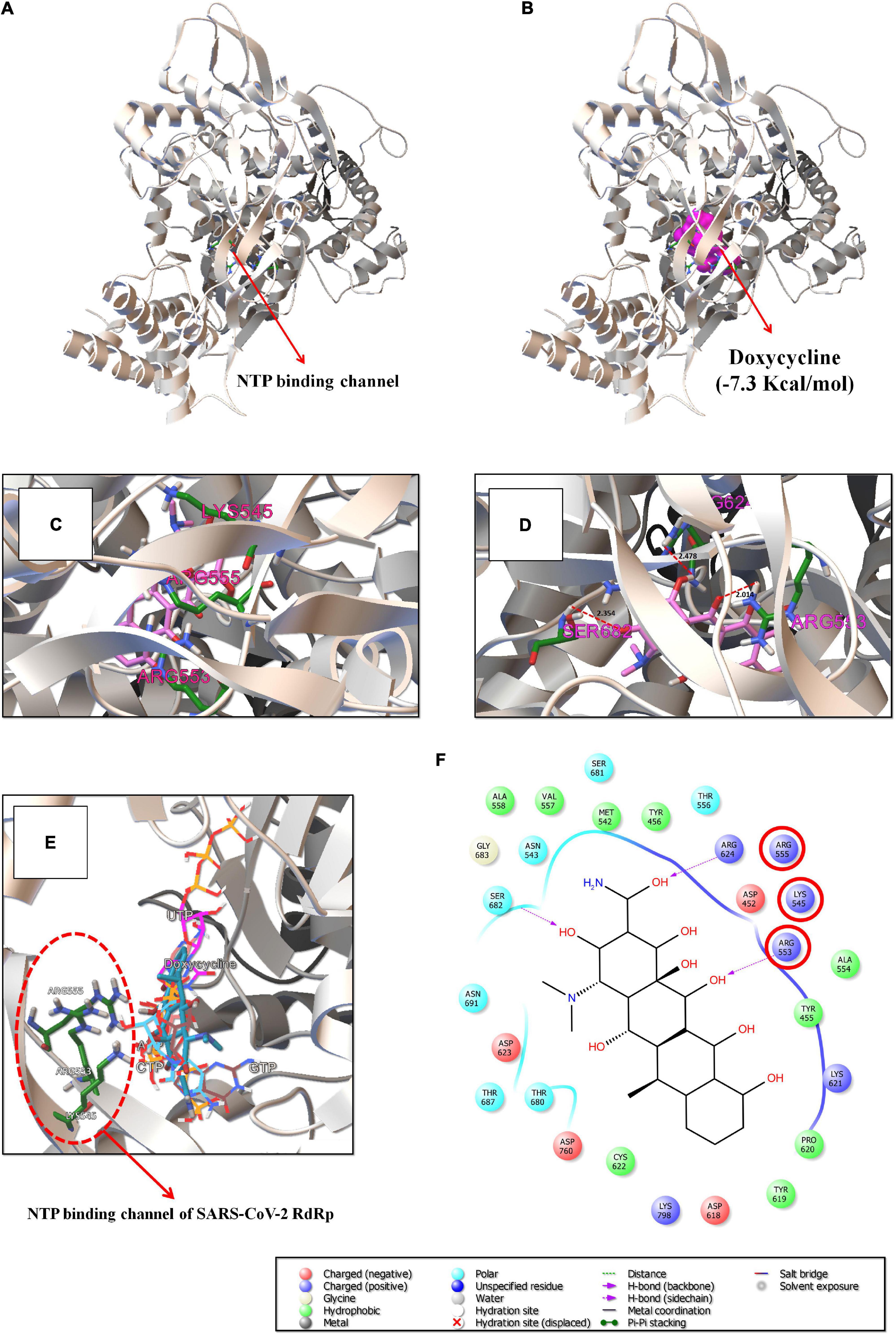
Figure 2. Panel (A) shows the position of the NTP binding channel of SARS-CoV-2 (set of hydrophilic residues such as Lys545, Arg553, and Arg555). Panels (B,C) indicate the overview and close-up view of the binding interaction of doxycycline (–7.3 Kcal/mol) with the NTP binding channel of SARS-CoV-2. Panel (D) indicates the hydrogen bond formation of doxycycline with Arg553, Arg624, and Ser682 residues of SARS-CoV-2 RdRp at 2.014 Å, 2.478 Å, and 2.354 Å distances. Panel (E) indicates the antagonistic action of doxycycline on the binding sites of the parental-nucleotides of SARS-CoV-2 RdRp. Panel (F) demonstrates the interacted amino acid residues of SARS-CoV-2 RdRp with doxycycline.
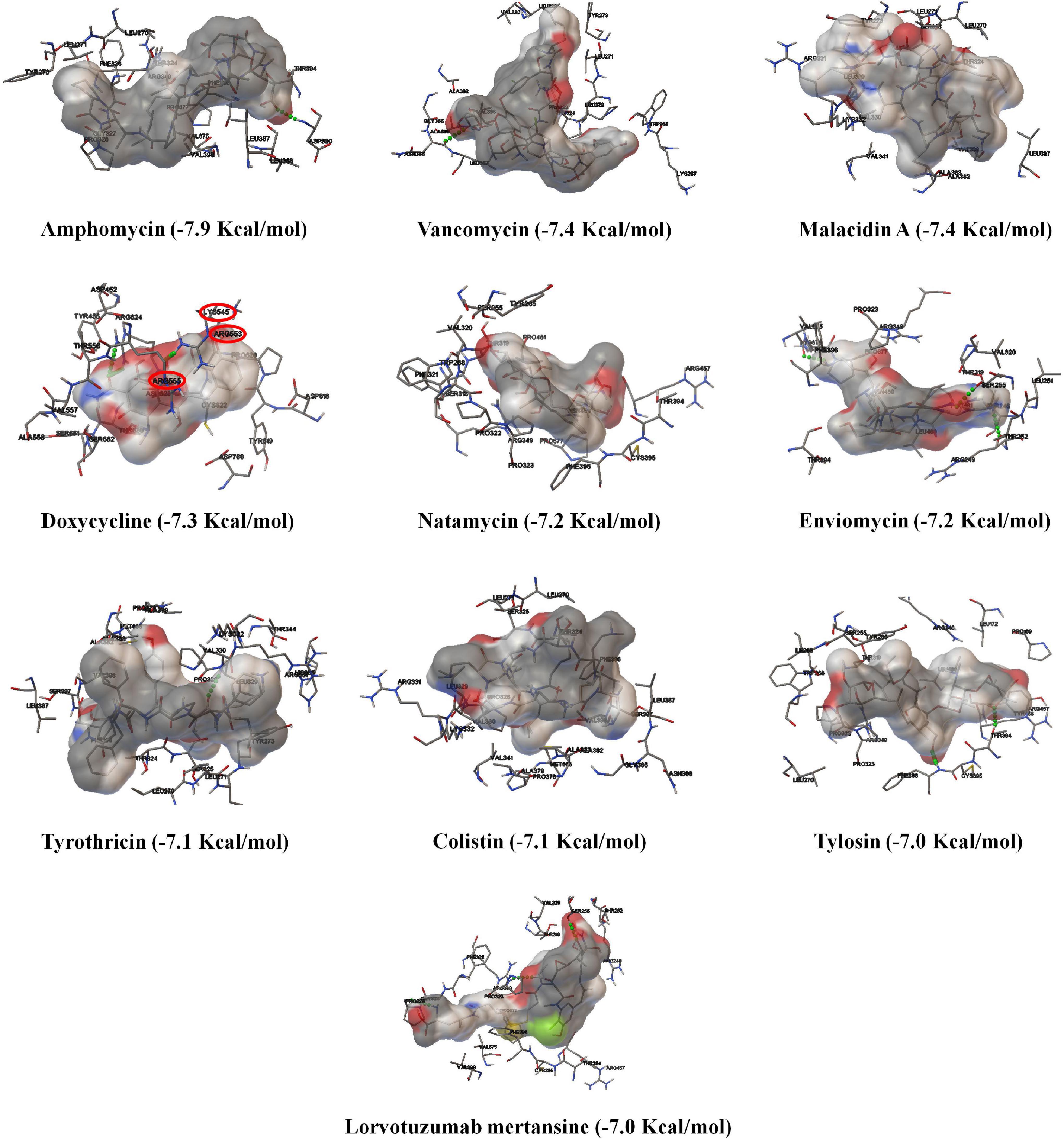
Figure 3. Schematic representation of mode of action of doxycycline against SARS-CoV-2. The binding patterns and aminoacid interactions of the best scoring antibacterial drugs with SARS-CoV-2 RdRp. The red circle-marking indicates the interaction with the active site (NTP binding channel) of SARS-CoV-2 RdRp.
As shown in Figure 3, doxycycline strongly builds nine hydrophobic bonds (Ala558, Val557, Met542, Tyr456, Ala554, Tyr455, Pro620, Tyr619, and Cys622), seven polar bonds (Asn691, Thr687, Thr680, Ser682, Asn543, Ser681, and Thr556), four negative-charged bonds (Asp623, Asp760, Asp618, and Asp452), and six unspecified bonds with Arg553, Lys545, Arg555, Lys621, Lys798, and Arg624. Importantly, doxycycline creates three hydrogen bond interactions with Arg553, Arg624, and Ser682 residues at 2.014 Å, 2.478 Å, and 2.354 Å distance, respectively (Figure 3). Based on the obtained results, we anticipate that the existing antibacterial drug, doxycycline, will interrupt the NTP entry channel and arrest viral replication, as is the case with remdesivir triphosphate, a known anti-COVID-19 agent (Eastman et al., 2020).
Molecular Dynamics Studies of Structural Stability
The structural stability of RdRp-Doxycycline complex was analyzed using root mean square deviation (RMSD), root mean square fluctuation (RMSF), and hydrogen bond (H-bonds) interactions. The RMSD and RMSF plots of the RdRp-Doxycycline complex revealed deviation and residual fluctuation values in the range of 0.25–0.35 nm and 0.1–0.55 nm, respectively, suggesting the RdRp-Doxycycline complex was very stable during the dynamic periods. The RMSD profile reveals that the structural behavior of RdRp-Doxycycline maintains their structural integrity during the 100 ns simulation (Figure 4A). The RMSF profile also revealed the structural stability of the RdRp-Doxycycline without major structural deviation (Figure 4B). Furthermore, the H-bonds plots showed that the complex has five H-bond interactions in the active site pocket (Figure 4C). These H-bonds were found to constantly interact with catalytic residues such as Arg553, Asp761, Asp760, Lys621, and Arg624, suggesting that doxycycline could inhibit the function of SARS-CoV-2 RdRp (Table 1).
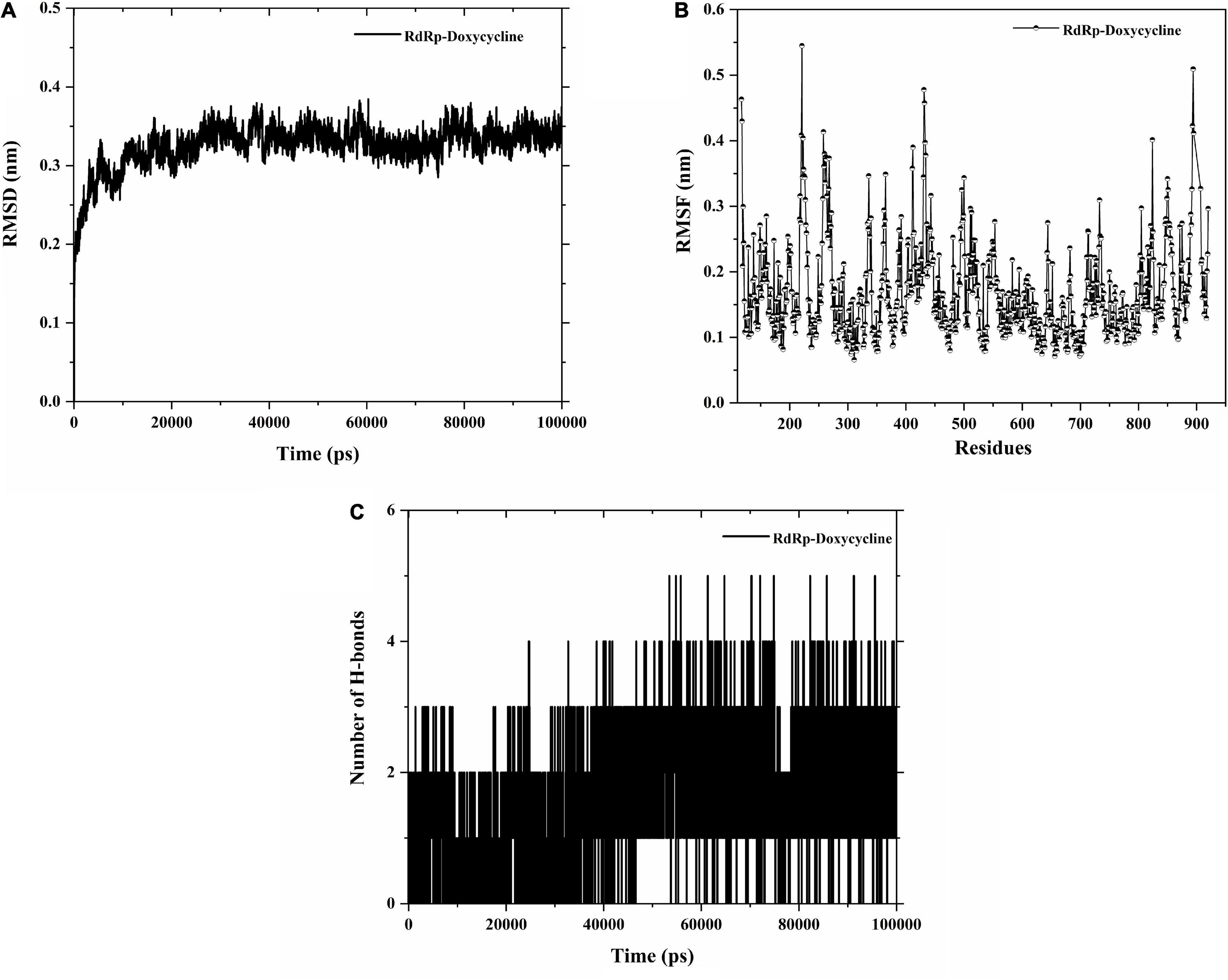
Figure 4. Panels (A,B) show the RMSD and RMSF profiling of the RdRp-Doxycycline complex during 100 ns simulation. Panel (C) indicates the hydrogen bond routes of the RdRp-Doxycycline complex during 100 ns simulation.
MM-PBSA Binding Free Energy Calculation
The binding-free energy calculation also helps to understand the stability of protein-ligand complexes in terms of affinity during molecular dynamics. It calculates major interaction energies such as electrostatic, Van der Waals, solvent accessible surface area, and total energy of the protein-ligand complexes. In Table 2, the RdRp-Doxycycline complex showed higher electrostatic interaction energy (−216.693 kj/mol), Van der Waals energy (−469.363 kj/mol), SASA energy (−17.681 kj/mol), and total binding free energy (−163.980 kj/mol). Significantly, Van der Waals energy is more favorable in the RdRp-Doxycycline complex due to the desolvation effect of active site residues in the cavity, while the electrostatic interaction energy showed relatively lesser affinity due to the attractive and repulsive parts of the complex. These two interaction energies are important to estimate the stable conformation of the RdRp-Doxycycline complex. Similarly, solvent accessible surface area (SASA) calculates the cavitations, dispersion, and repulsion energies of the RdRp-Doxycycline complex suggesting that the complex is stable during the dynamics. Hence, the hydrophobic, polar and negative charged interactions are so much important for RdRp-drug binding. Overall, the obtained results reveal that doxycycline has a higher binding affinity toward the complex formation with the therapeutic protein of SARS-CoV-2.
In vitro Antiviral Activity of Doxycycline Against SARS-CoV-2
The antiviral activity was evaluated using an in vitro experiment with the clinical isolates (IHUMI-3 and IHUMI-6) of SARS-CoV-2. The results revealed that the tested antibacterial drug (doxycycline) showed efficient antiviral activity against the clinical isolates (IHUMI-3 and IHUMI-6) of SARS-CoV-2 at lower concentration (Table 3). The results showed that the dose-dependent responses were observed for doxycycline, chloroquine, and remdesivir against SARS-CoV-2, as shown in Figure 5. The median effective concentration (EC50) and 90% effective concentration (EC90) of doxycycline against the IHUMI-3 strain are 5.8 ± 1.6 μM and 21.7 ± 5.9 μM, respectively, at 0.8 MOI. Consistently, the EC50 and EC90 of doxycycline against IHUMI-6 are 35.4 ± 12.4 μM and 361 ± 69 μM at 0.8 MOI. The results obtained reveal that the antiviral behavior of doxycycline against SARS-CoV-2 was strain-dependent. Overall, the in vitro data strongly validated the in silico result and proved the antiviral activity of doxycycline against SARS-CoV-2. Doxycycline (35.4 μM) was much less effective than remdesivir (4.3 μM) and chloroquine (8.7 μM) against IUHMI-6. There was no difference between in vitro responses to doxycycline, remdesivir, or chloroquine against IHUMI-3 (p = 0.129, Kruskal–Wallis test). Simultaneously, other top binding-compounds, such as amphomycin, vancomycin, malacidin A, natamycin, enviomycin, tyrothricin, colistin, tylosin, and lorvotuzumab mertansine were found to be ineffective against SARS-CoV-2.
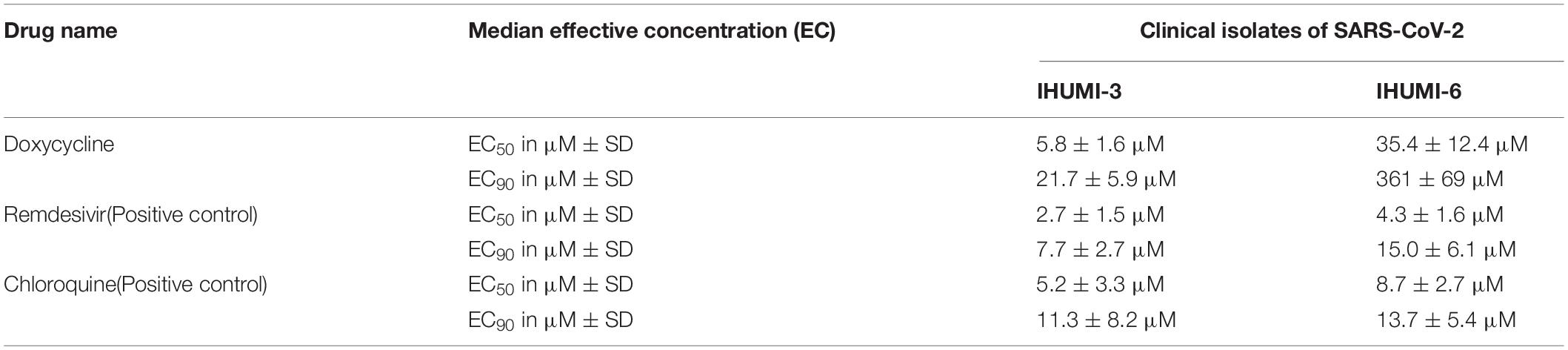
Table 3. In vitro antiviral activity of tested antibacterial drugs against the clinical isolates of SARS-CoV-2.
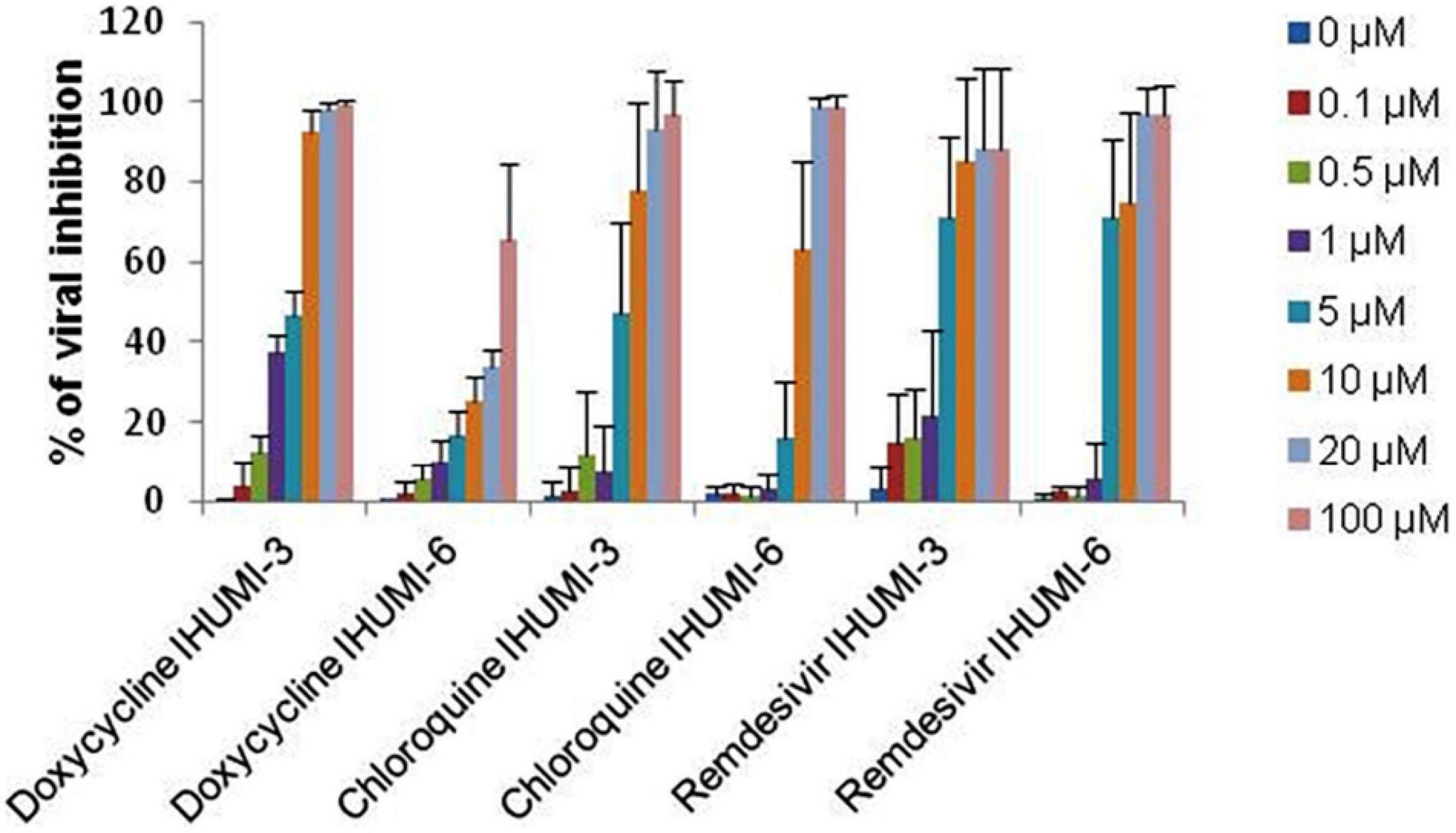
Figure 5. Anti-SARS-CoV-2 activity of doxycycline, chloroquine and remdesivir in % of antiviral inhibition on IHUMI-3 and IHUMI-6 clinically-isolated strains (error bars represent standard deviation of 7–10 independent experiments).
Furthermore, the effects of doxycycline, chloroquine, or remdesivir on entry and post-entry of SARS-CoV-2 was evaluated using IHUMI-3 strain at a concentration of 10 μM. The result shows that doxycycline well interacted at both entry and post-entry stages of SARS-CoV-2 infection in Vero E6 cells, as chloroquine did (Figure 6). In reverse, remdesivir, which is an anti-SARS-CoV-2 drug, interacted only at post-entry stage. Hence, the in vitro results strongly suggest that the doxycycline could be a potent antiviral compound for the development of therapeutic medication against COVID-19 as like chloroquine and remdesivir.
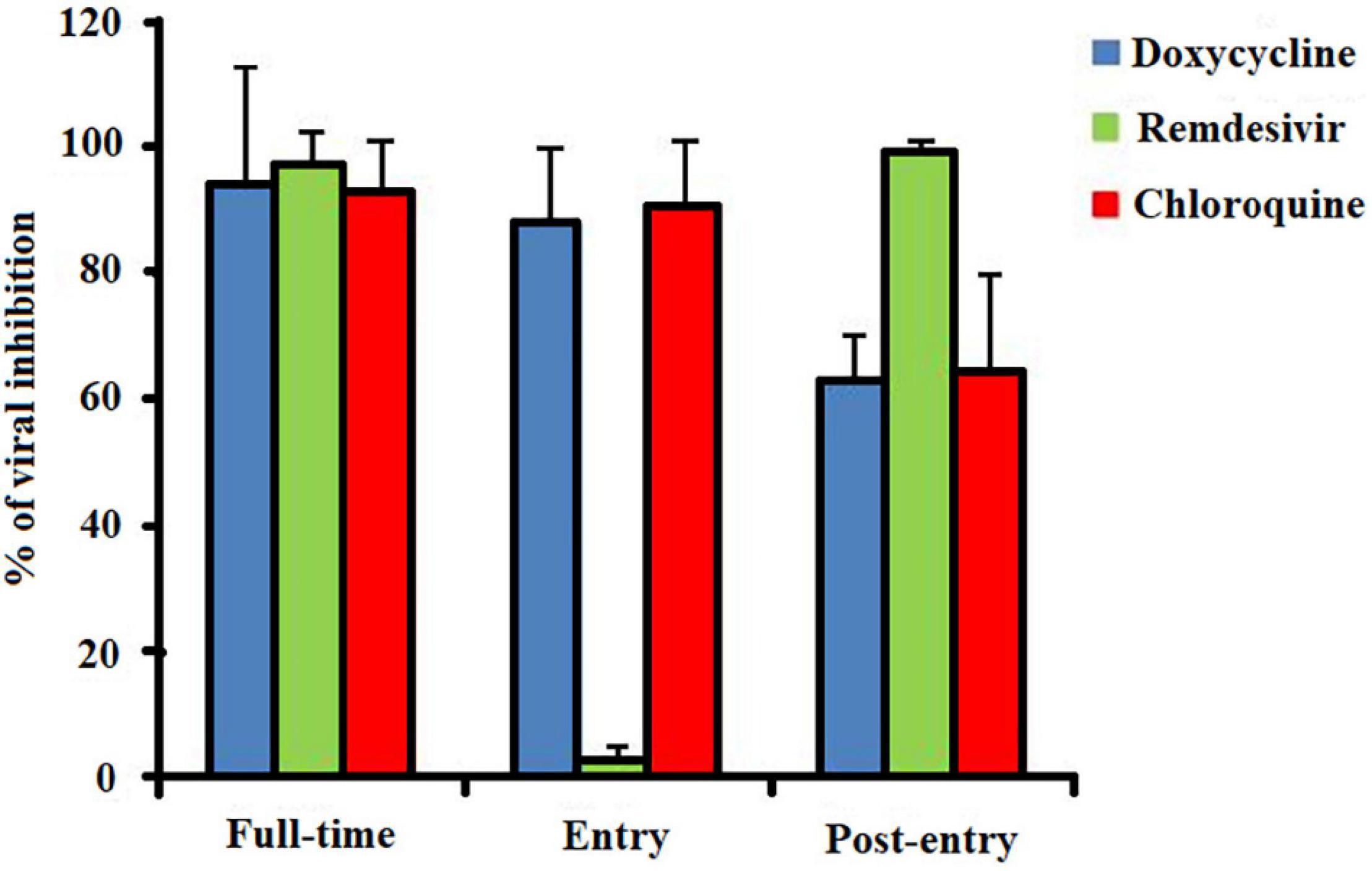
Figure 6. In vitro antiviral activity of doxycycline, chloroquine, and remdesivir at 10 μM against the SARS-CoV-2 IHUMI-3 strain. For the “full-time” treatment, Vero E6 cells were infected with the IHUMI-3 strain for 48 h after pre-incubation of the cells with one of the three drugs for 4 h. For the “entry” treatment, the cells were infected for 2 h after pre-incubation for 4 h and then the virus-drug mixture was replaced with fresh medium maintained for 46 h. For the “post-entry” treatment, the cells were infected for 2 h and then incubated with drug for 46 h.
Discussion
Several antiviral agents have been assessed against viral proteases, polymerases, and entry proteins of SARS-CoV-2 for treating COVID-19 (Rosa and Santos, 2020). The described anti-COVID-19 drugs are largely inhibitors for 3CLpro or RdRp. For instance, in remdesivir, a prodrug targets budding viral-RNA by blocking the activity of SARS-CoV-2 RdRp, which leads to the arrest of RNA synthesis (Elfiky, 2020). Likewise, favipiravir and ribavirin have also demonstrated their potential against COVID-19 (Cai et al., 2020). Owing to the crucial role of viral proteins, SARS-CoV-2 3CLpro and RdRp have been seen as a hopeful therapeutic approach to treating COVID-19 (Ahmed et al., 2020; Wu et al., 2020).
During the development of drugs against COVID-19, SARS-CoV-2 RdRp has been considered as an important therapeutic target owing to the central role it plays in RNA synthesis from the RNA-template during replication and transcription (Elfiky, 2020). Therefore, SARS-CoV-2 RdRp is a key when it comes to nucleotide analog-drugs such as remdesivir, exhibits positive results for drug-repurposing treatment against COVID-19 (Choy et al., 2020). Further, nucleotide analog-based antiviral drugs were unable to demonstrate significant reverse effects on humans (Dong et al., 2020). The RdRp-complex is activated through polymerase motifs A to G, located in the 549–776 regions, which relate to RNA-mediated 5′–3′ polymerase activity. Similarly to other RdRp, the NTP entry channel and budding strand assemble at a central hollow where RdRp-motifs mediate RNA-template based replication by the NTP binding site located at Lys545, Arg553, and Arg555 (Gao et al., 2020). The nucleotide analogs, namely favipiravir and remdesivir, arrest NTP binding, and thereby showed their antiviral activity against COVID-19 (Gordon et al., 2020).
In the current study, the in silico results obtained reveal that doxycycline has the potential to interrupt with the NTP entry channel of SARS-CoV-2 RdRp (Figure 3). Furthermore, the binding site of doxycycline with the NTP entry channel of RdRp was confirmed by re-docking with the SARS-CoV-2 RdRp-Nsp7-Nsp8 complex (PDB ID: 7AAP). The result also showed that doxycycline was able to interfere with NTP entry with low-binding energy (−10.5 Kcal/mol), and hence we believed to arrest the viral replication of SARS-CoV-2. In molecular simulation analysis, the RMSD and RMSF graphs illustrated no major fluctuation for the RdRp-Doxycycline complex (Figure 4). The structural stability of the complexes did not exceed 0.5 nm, suggesting that all the complexes were architecturally highly stable, indicating that the doxycycline could be active against SARS-CoV-2. Furthermore, the MM-PBSA calculation revealed that the RdRp-Doxycycline complex possesses virtuous affinities such as high-electrostatic interaction, Van der Waals interaction, and SASA energy toward to the catalytic domains of SARS-CoV-2 RdRp, leading to greater attention being paid to assessing the potential of doxycycline against SARS-CoV-2.
As expected, the in vitro experiment confirmed the antiviral activity of doxycycline against SARS-CoV-2 at low micromolar concentrations (Table 3). The obtained results revealed that doxycycline may have the potential to hinder the viral replication of both the IHUMI-3 and IHUMI-6 strains of SARS-CoV-2. The EC50 and EC90 values of doxycycline were found to be 5.8 ± 1.6 μM and 21.7 ± 5.9 μM against the IHUMI-3 strain at an MOI of 0.8, in coherence with previous data (Gendrot et al., 2020). Doxycycline is as effective in vitro as remdesivir or chloroquine against IHUMI-3 strain (p = 0.129, Kruskal–Wallis test). However, doxycycline is less significantly active against the IHUMI-6 strain of SARS-CoV-2 (EC50 = 35.4 ± 12.4 μM and EC90 = 361 ± 69). Moreover, the doxycycline inhibited the viral load in vitro at both on entry and after viral entry of SARS-CoV-2 (Figure 6). Based on in silico and in vitro experiments, the current study suggests that doxycycline could be a feasible drug candidate for a potential COVID-19 treatment.
The lack of in vivo study or human trial to address the therapeutic action of doxycycline against COVID-19 is an impeding limitation of this study. As a part of drug repurposing actions, doxycycline was known to have in vitro activity against SARS-CoV-2 (Gendrot et al., 2020). The possible therapeutic action of doxycycline is due to the pleiotropic effects against the general pathways involved in viral infection, replication, and associated over expression of the zinc finger antiviral protein, which binds to viral messenger RNAs and inhibits translation of viral RNAs (Malek et al., 2020). Doxycycline has anti-inflammatory, antibacterial, and possibly antiviral effects, and so was believed as potential treatments for COVID-19. Malek and Granwehr (2021) have reported doxycyline as an alternative to azithromycin in elderly patients for the treatment of COVID-19. In the majority of cases, early dose of doxycycline treatment was related with improved clinical outcomes, decreased hospitalizations, and decreased mortality. However, larger randomized control trials are needed to evaluate the outcome of doxycycline treatment in moderate to severe COVID-19 infections (Alam et al., 2020). Yates et al. (2020) also reported that the doxycycline resolved the symptoms of high-risk COVID-19-positive patients with comorbid pulmonary disease. Unfortunately, the randomized evaluation of COVID-19 therapy trial recently reported that the use of doxycycline treatment in patients hospitalized with severe COVID-19 condition had no significant clinical assistance (Butler et al., 2021). It may be due to the development of resistance as well as the emergence of the variants among SARS-CoV-2 strains. Our results also revealed that the in vitro antiviral potential of doxycycline was differed based on the strains of SARS-CoV-2. On the other hand, the patients with COVID-19 infection who received doxycycline with ivermectin recovered faster than those who received placebo, were less likely to progress to severe illness, and were much more likely to test negative for COVID-19 (Mahmud et al., 2021). Overall, the pilot study suggests that doxycycline might be valuable while combination with other drugs for the treatment of COVID-19.
Conclusion
Owing to its potential ability to target the therapeutic protein of SARS-CoV-2 in silico, doxycycline was predicted as the potent compound to arrest the viral replication through docking analysis. Molecular dynamics studies also revealed that the RdRp-doxycycline complex is architecturally highly stable and its complexes maintain close contact with their active catalytic domains. The MM-PBSA calculation also recommends that doxycycline has well-intentioned binding affinities with the catalytic domains of RdRp, suggesting that the doxycycline could be the potent antiviral compound against SARS-CoV-2. As predicted, doxycycline has the potential to hinder the viral replication of SARS-CoV-2 at low concentration. The in vitro results clearly demonstrate that doxycycline effectively arrested the viral replication of both IHUMI-3 and IHUMI-6 strains SARS-CoV-2 at low concentrations. Based on the results, the present study suggests that doxycycline could be very useful in combination with other anti-SARS-CoV-2 drugs for the treatment of COVID-19. However, further in vivo studies with animal models, followed by human trials, are required to authenticate the clinical effectiveness of doxycycline against COVID-19.
Data Availability Statement
The original contributions presented in the study are included in the article/Supplementary Material, further inquiries can be directed to the corresponding author/s.
Author Contributions
RA contributed to conceptualization, performed the in silico experiments, data analysis, and writing-original draft. GA performed the in silico experiments. MG, OD, IF, and JM performed the in vitro experiments with SARS-CoV-2. RM contributed in molecular simulation. JJ contributed in reviewing-original draft and data analysis. SP contributed in reviewing-original draft. BP and AR contributed in supervision, data analysis, and reviewing-original draft. All authors contributed to the article and approved the submitted version.
Funding
RA acknowledge financial support from RUSA, MHRD, Government of India [F. 24-51/2014-U, Policy (TN Multi-Gen), Dept. of Edn, GOI] in the form of RUSA-2.0 Fellowship. The evaluation of the in vitro activity was supported by the Institut Hospitalo-Universitaire (IHU) Méditerranée Infection and the French National Research Agency under the “Investissements d’avenir” program (Ref: ANR-10-IAHU-03).
Conflict of Interest
The authors declare that the research was conducted in the absence of any commercial or financial relationships that could be construed as a potential conflict of interest.
Publisher’s Note
All claims expressed in this article are solely those of the authors and do not necessarily represent those of their affiliated organizations, or those of the publisher, the editors and the reviewers. Any product that may be evaluated in this article, or claim that may be made by its manufacturer, is not guaranteed or endorsed by the publisher.
Acknowledgments
RA sincerely express thanks to Hua Li, HUST, Wuhan, China for providing the modeled structure of SARS-CoV-2 RdRp. RA also acknowledge Ragupathi Thennarasu, DBT, ALU for constant support and caring throughout the study.
Supplementary Material
The Supplementary Material for this article can be found online at: https://www.frontiersin.org/articles/10.3389/fmicb.2022.757418/full#supplementary-material
Footnotes
References
Abd El-Aziz, T. M., and Stockand, J. D. (2020). Recent progress and challenges in drug development against COVID-19 coronavirus (SARS-CoV-2)-an update on the status. Infect. Genet. Evol. 83:104327. doi: 10.1016/j.meegid.2020.104327Get
Ahmed, S. F., Quadeer, A. A., and McKay, M. R. (2020). Preliminary identification of potential vaccine targets for the COVID-19 coronavirus (SARS-CoV-2) based on SARS-CoV immunological studies. Viruses 12:254. doi: 10.3390/v12030254
Alam, M. M., Mahmud, S., Rahman, M. M., Simpson, J., Aggarwal, S., and Ahmed, Z. (2020). Clinical outcomes of early treatment with doxycycline for 89 high-risk COVID-19 patients in long-term care facilities in New York. Cureus 12:e9658. doi: 10.7759/cureus.9658
Alexpandi, R., De Mesquita, J. F., Pandian, S. K., and Ravi, A. V. (2020). Quinolines-based SARS-CoV-2 3CLpro and RdRp inhibitors and spike-RBD-ACE2 inhibitor for drug-repurposing against COVID-19: an in silico analysis. Front. Microbiol. 11:1796. doi: 10.3389/fmicb.2020.01796
Alexpandi, R., Prasanth, M. I, Ravi, A. V., Balamurugan, K., Durgadevi, R., Srinivasan, R., et al. (2019). Protective effect of neglected plant Diplocyclos palmatus on quorum sensing mediated infection of Serratia marcescens and UV-A induced photoaging in model Caenorhabditis elegans. J. Photochem. Photobiol. B. Biol. 201:111637. doi: 10.1016/j.jphotobiol.2019.111637
Andreani, J., Le Bideau, M., Duflot, I., Jardot, P., Rolland, C., Boxberger, M., et al. (2020). In vitro testing of combined hydroxychloroquine and azithromycin on SARS-CoV-2 shows synergistic effect. Microb. Pathog 145:104228. doi: 10.1016/j.micpath.2020.104228Get
Balasubramaniam, B., Alexpandi, R., and Darjily, D. R. (2019). Exploration of the optimized parameters for bioactive prodigiosin mass production and its biomedical applications in vitro as well as in silico. Biocatal. Agric. Biotechnol. 22:101385. doi: 10.1016/j.bcab.2019.101385
Butler, C. C., Yu, L. M., Dorward, J., Gbinigie, O., Hayward, G., Saville, B. R., et al. (2021). Doxycycline for community treatment of suspected COVID-19 in people at high risk of adverse outcomes in the UK (PRINCIPLE): a randomised, controlled, open-label, adaptive platform trial. Lancet Respir. Med. 9, 1010–1020. doi: 10.1016/S2213-2600(21)00310-6Get
Cai, Q., Yang, M., Liu, D., Chen, J., Shu, D., Xia, J., et al. (2020). Experimental treatment with favipiravir for COVID-19: an open-label control study. Engineering. 6, 1192–1198. doi: 10.1016/j.eng.2020.03.007
Cheng, M. P., Lee, T. C., Tan, D. H., and Murthy, S. (2020). Generating randomized trial evidence to optimize treatment in the COVID-19 pandemic. Cmaj. 192, E405–407. doi: 10.1503/cmaj.200438
Choubey, S. K., Nachiappan, M., Richard, M., Chitra, J. P., and Jeyakanthan, J. (2020). Structural and functional insights of STAT2-NS5 interaction for the identification of NS5 antagonist - An approach for restoring interferon signaling. Comput. Biol. Chem. 88:107332. doi: 10.1016/j.compbiolchem.2020.107332
Choy, K. T., Wong, A. Y. L., Kaewpreedee, P., Sia, S. F., Chen, D., Hui, K. P. Y., et al. (2020). Remdesivir, lopinavir, emetine, and homoharringtonine inhibit SARS-CoV-2 replication in vitro. Antivir. Res 178:104786. doi: 10.1016/j.antiviral.2020.104786Get
Cimolai, N. (2020). Potentially repurposing adamantanes for COVID-19. J. Med. Virol. 92, 531–532. doi: 10.1002/jmv.25752
Corman, V. M., Landt, O., Kaiser, M., Molenkamp, R., Meijer, A., Chu, D. K. W., et al. (2020). Detection of 2019 novel coronavirus (2019-nCoV) by real-time RT-PCR. Euro. Surveill. 25:2000045. doi: 10.2807/1560-7917.ES.2020.25.3.2000045
Dong, L., Hu, S., and Gao, J. (2020). Discovering drugs to treat coronavirus disease 2019 (COVID-19). Drug. Discov. Ther. 14, 58–60. doi: 10.5582/ddt.2020.01012
Eastman, R. T., Roth, J. S., Brimacombe, K. R., Simeonov, A., Shen, M., Patnaik, S., et al. (2020). Remdesivir: a review of its discovery and development leading to emergency use authorization for treatment of COVID-19. ACS Cent. Sci. 6, 672–683. doi: 10.1021/acscentsci.0c00489
Elfiky, A. (2020). Ribavirin, Remdesivir, Sofosbuvir, Galidesivir, and Tenofovir against SARS-CoV-2 RNA dependent RNA polymerase (RdRp): a molecular docking study. Life. Sci. 253:117592. doi: 10.1016/j.lfs.2020.117592
Gao, Y., Yan, L., Huang, Y., Liu, F., Zhao, Y., Cao, L., et al. (2020). Structure of the RNA-dependent RNA polymerase from COVID-19 virus. Science 368, 779–782. doi: 10.1126/science.abb7498
Gautret, P., Lagier, J. C., Parola, P., Meddeb, L., Mailhe, M., Doudier, B., et al. (2020). Hydroxychloroquine and azithromycin as a treatment of COVID-19: results of an open-label non-randomized clinical trial. Int. J. Antimicrob. Agents. 56:105949. doi: 10.1016/j.ijantimicag.2020.105949
Gendrot, M., Andreani, J., Jardot, P., Hutter, S., Delandre, O., Boxberger, M., et al. (2020). In vitro antiviral activity of doxycycline against SARS-CoV-2. Molecules. 25, 5064. doi: 10.3390/molecules25215064
Gordon, C. J., Tchesnokov, E. P., Woolner, E., Perry, J. K., Feng, J. Y., Porter, D. P., et al. (2020). Remdesivir is a direct-acting antiviral that inhibits RNA-dependent RNA polymerase from severe acute respiratory syndrome coronavirus 2 with high potency. J. Biol. Chem. 295, 6785–6797. doi: 10.1074/jbc.RA120.013679
Gostic, K., Gomez, A. C., Mummah, R. O., Kucharski, A. J., and Lloyd-Smith, J. O. (2020). Estimated effectiveness of symptom and risk screening to prevent the spread of COVID-19. Elife. 9:55570. doi: 10.7554/eLife.55570.sa2
Li, S., Wang, Y., Xue, J., Zhao, N., and Zhu, T. (2020). The impact of COVID-19 epidemic declaration on psychological consequences: a study on active Weibo users. Int. J. Environ. Res. Public Health. 17:2032. doi: 10.3390/ijerph17062032
Mahmud, R., Rahman, M. M., Alam, I., Ahmed, K. G., Kabir, A. H., Sayeed, S. J., et al. (2021). Ivermectin in combination with doxycycline for treating COVID-19 symptoms: a randomized trial. J. Int. Med. Res. 49:03000605211013550. doi: 10.1177/03000605211013550
Malek, A. E., and Granwehr, B. P. (2021). Doxycycline as an alternative to azithromycin in elderly patients. Int. J. Antimicrob. Agents. 57:106168. doi: 10.1016/j.ijantimicag.2020.106168
Malek, A. E., Granwehr, B. P., and Kontoyiannis, D. P. (2020). Doxycycline as a potential partner of COVID-19 therapies. IDCases. 21:e00864. doi: 10.1016/j.idcr.2020.e00864
Mariadasse, R., Choubey, S. K., and Jeyakanthan, J. (2020). Insights into exogenous tryptophan-mediated allosteric communication and helical transition of trp protein for transcription regulation. J. Chem. Inf. Model. 60, 175–191. doi: 10.1021/acs.jcim.9b00755
Menni, C., Valdes, A. M., Freidin, M. B., Sudre, C. H., Nguyen, L. H., Drew, D. A., et al. (2020). Real-time tracking of self-reported symptoms to predict potential COVID-19. Nat. Med. 26, 1037–1040. doi: 10.1038/s41591-020-0916-2
Owen, D. R., Allerton, C. M., Anderson, A. S., Aschenbrenner, L., Avery, M., Berritt, S., et al. (2021). An oral SARS-CoV-2 Mpro inhibitor clinical candidate for the treatment of COVID-19. Science. 374, 1586–1593. doi: 10.1126/science.abl4784
Pawar, A. Y. (2020). Combating devastating COVID-19 by drug repurposing. Int. J. Antimicrob. Agents 56:105984. doi: 10.1016/j.ijantimicag.2020.105984
Phadke, M., and Saunik, S. (2020). COVID-19 treatment by repurposing drugs until the vaccine is in sight. Drug. Dev. Res. 81, 541–543. doi: 10.1002/ddr.21666
Rafiq, D., Batool, A., and Bazaz, M. A. (2020). Three months of COVID-19: a systematic review and meta-analysis. Rev. Med. Virol. 30:e2113. doi: 10.1002/rmv.2113
Rathnayake, A. D., Zheng, J., Kim, Y., Perera, K. D., Mackin, S., Meyerholz, D. K., et al. (2020). 3C-like protease inhibitors block coronavirus replication in vitro and improve survival in MERS-CoV–infected mice. Sci. Transl. Med. 12:eabc5332. doi: 10.1126/scitranslmed.abc5332
Rosa, S. G. V., and Santos, W. C. (2020). Clinical trials on drug repositioning for COVID-19 treatment. Rev. Panam. Salud Públ. 44:e40. doi: 10.26633/RPSP.2020.40
Shah, B., Modi, P., and Sagar, S. R. (2020). In silico studies on therapeutic agents for COVID-19: Drug repurposing approach. Life Sci. 252:117652. doi: 10.1016/j.lfs.2020.117652
Wu, C., Liu, Y., Yang, Y., Zhang, P., Zhong, W., Wang, Y., et al. (2020). Analysis of therapeutic targets for SARS-CoV-2 and discovery of potential drugs by computational methods. Acta. Pharma. Sin. B. 10, 766–788. doi: 10.1016/j.apsb.2020.02.008
Yates, P. A., Newman, S. A., Oshry, L. J., Glassman, R. H., Leone, A. M., and Reichel, E. (2020). Doxycycline treatment of high-risk COVID-19-positive patients with comorbid pulmonary disease. Ther. Adv. Respir. Dis. 14:1753466620951053. doi: 10.1177/1753466620951053
Zhang, Y. Z., and Holmes, E. C. (2020). A genomic perspective on the origin and emergence of SARS-CoV-2. Cell. 181, 223–227. doi: 10.1016/j.cell.2020.03.035
Keywords: COVID-19, drug repurposing, doxycycline, SARS-CoV-2, in silico, in vitro
Citation: Alexpandi R, Gendrot M, Abirami G, Delandre O, Fonta I, Mosnier J, Mariadasse R, Jeyakanthan J, Pandian SK, Pradines B and Ravi AV (2022) Repurposing of Doxycycline to Hinder the Viral Replication of SARS-CoV-2: From in silico to in vitro Validation. Front. Microbiol. 13:757418. doi: 10.3389/fmicb.2022.757418
Received: 12 August 2021; Accepted: 11 March 2022;
Published: 04 May 2022.
Edited by:
Nejat Duzgunes, University of the Pacific, United StatesReviewed by:
Debmalya Barh, Institute of Integrative Omics and Applied Biotechnology (IIOAB), IndiaAlexandre Malek, Ochsner LSU Health, United States
Copyright © 2022 Alexpandi, Gendrot, Abirami, Delandre, Fonta, Mosnier, Mariadasse, Jeyakanthan, Pandian, Pradines and Ravi. This is an open-access article distributed under the terms of the Creative Commons Attribution License (CC BY). The use, distribution or reproduction in other forums is permitted, provided the original author(s) and the copyright owner(s) are credited and that the original publication in this journal is cited, in accordance with accepted academic practice. No use, distribution or reproduction is permitted which does not comply with these terms.
*Correspondence: Bruno Pradines, bruno.pradines@gmail.com; Arumugam Veera Ravi, aveeraravi@rediffmail.com